Current Projects
- DFG project: CarboDeg – Investigating the complex interplay between structure and stability of carbon materials in electrochemical systems using the vanadium redox-flow battery as an example, 10/2023-09/2026
- DFG project: MicroSpec – Micro-Spectroelectrochemical investigations of the incorporation of iron ions in nickel oxide and its effect on the oxygen evolution activity during alkaline water electrolysis (PI: Dr. Matthias Steimecke), 02/2024 – 01/2027
- Structure-activity relationships in carbon-based electrocatalysts
DFG-Project: CarboDeg - Investigating the complex interplay between structure and stability of carbon materials in electrochemical systems using the vanadium redox-flow battery as an example
Considering the continuous growth of fluctuating renewable energies, the storage of electricity becomes more and more important. Electrochemical energy storage systems can significantly contribute to the available storage capacity, with vanadium redox flow batteries (VRFBs), which store energy in redox couples in the electrolyte solution rather than in the electrodes, being particularly discussed for stationary applications. In addition to parameters such as efficiency and energy or power density, the long-term stability of the individual components in such VRFB is also of high relevance for economic application.
Being the location, where the respective redox reactions take place, the carbon-based electrodes are of major importance. Since their degradation can have a large variety of causes, complex degradation mechanisms may result, which need to be systematically analysed to develop stable materials. In this project, carbon-based electrode materials of increasing complexity and with different surface properties are tested for their chemical and electrochemical stability under a broad range of experimental conditions. Various structural characterisation methods, such as Raman spectroscopy, X-ray photoelectron spectroscopy (XPS) or X-ray diffraction (XRD), as well as electrochemical methods, e.g. cyclic voltammetry (CV) or electrochemical impedance spectroscopy (EIS), are used to investigate the different materials before and after long-term electrochemical treatment. The underlying factors influencing the stability of carbon-based electrodes shall be identified, and the gained knowledge could be used as a guideline for developing long-lasting components. In situ techniques, especially those based on Raman and IR spectroscopy, as well as coupled microelectrochemical methods also play a crucial role in our research.
![Figure 1: Schematic representation of a VRFB including an illustration of selected electrode properties.[1]](/im/1709196857_1600_00_200.jpg)
Figure 1: Schematic representation of a VRFB including an illustration of selected electrode properties.[1]
[1] B. M. Sivakumar, V. Prabhakaran, K. Duanmu, E. Thomsen, B. Berland, N. Gomez, D. Reed, V. Murugesan, ACS Appl. Energy Mater. 2021, 4, 6074.
DFG project: MicroSpec – Micro-Spectroelectrochemical investigations of the incorporation of iron ions in nickel oxide and its effect on the oxygen evolution activity during alkaline water electrolysis (PI: Dr. Matthias Steimecke), 02/2024 – 01/2027
The efficient storage of renewable electricity generated from wind and solar power is one of the key challenges of our time. In addition to accumulators for feeding electricity from producers and consumers into and out of the grid, electrolysis processes can provide important raw materials for the chemical industry. Hydrogen is the central molecule targeted by current developments. In addition to acidic membrane electrolysis (PEM), which relies on stable and efficient but also rare and expensive materials, alkaline water electrolysis plays an important role in the necessary transformation of the basic material supply from fossil fuels to renewable energies. In recent years, decisive progress has been made in the field of alkaline membranes, with alkaline membrane electrolysis (AEM) making a decisive contribution. Electrode materials consisting of inexpensive and readily available transition metal compounds can be used here. However, there is a lack of understanding and a need for optimization, which makes further research necessary, especially for the side of the kinetically strongly inhibited oxygen evolution reaction (OER). The nickel-iron double hydroxide (NiFe-LDH) is of particular importance here, as it has the lowest losses (overvoltage).
In this project, new nickel oxide-based catalysts for the oxygen evolution reaction are to be investigated. The central idea of the project is to combine material knowledge of high-temperature nickel oxides and method development of spatially resolved electrochemistry and in situ spectroscopy. On the one hand, powdered nickel oxides treated at different temperatures with different iron contents are to be comprehensively investigated in order to develop novel materials with high activity and simultaneous stability for electrochemical oxygen evolution. The approach of using nickel oxide-based materials instead of hydroxide-based materials is based on our own results, in which high-temperature nickel oxide showed comparable activity with significantly improved stability compared to hydroxide. On the other hand, the combined in situ Raman microscopy and scanning electrochemical microscopy (SECM) will be further developed in this project using novel approaches. Finally, this setup will be used for the microelectrochemical and spectroscopic investigation of nickel (iron) oxide electrodes and provide new insights into activity-structure relationships and causes of deactivation and stability. Combined with the material knowledge, a deeper understanding of the dynamics of nickel-based electrocatalysts with regard to structural changes should be obtained and thus provide new approaches for more stable OER catalysts in alkaline electrolysis.
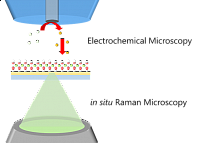
DFG MicroSpec
Electrochemical oxidation of small organic molecules: fuel cells and beyond
The electrochemical oxidation of small organic molecules is an active field of research since decades. Initially, a focus was on fuel cell-relevant reactions like methanol or ethylene glycol oxidation. In recent times, the focus of researchers however shifts towards applying oxidation reactions as alternative anode reactions in electrolysis to produce hydrogen. The idea is to replace the energy-demanding oxygen evolution reaction of the water electrolysis by a reaction showing a less positive potential and/or lower overpotentials. The substance to be oxidised may either be a sacrificial agent, or be converted to so-called value-added products, i.e. substances of possible use for industrial applications. Degradation of pollutants through oxidation is discussed as well.
Our group currently investigates the oxidation of ethylene glycol and glycerol using noble metal catalysts like Pt and Au. In a joint project with Prof. Dra. Daniela Mezalira, FUSC, Brazil, supported Pt and Au nanoparticles are applied in glycerol electrooxidation. The effect of support composition (e.g., functionalised nanotubes, composites of carbon-based materials with oxides) on the electrocatalytic properties is studies. Currently, the focus is on activity of the materials in electrooxidation, however selectivity issues will be addressed in the future as well.
Besides catalyst development, one aim of our work is also to understand reaction pathways and the underlying mechanisms in the electro-oxidation of small organic molecules. For this purpose, we are using in situ-spectroscopic techniques. In the present case, in situ-infrared spectroscopy is particularly suited, since intermediates and products of such oxidations typically show defined and discernible IR spectra. In ideal cases, adsorbed reaction intermediates can be monitored as well, providing direct clues towards reaction mechanism. By combining spectroscopic and electrochemical experiment (so-called spectroelectrochemistry), potential-dependent oxidation behaviour can be visualized. These studies are accompanied by a thorough electrochemical investigation and may be complemented by other analytical techniques such as gas chromatography.
As an example, the below figure display in situ-infrared spectra of ethylene glycol (0.01 M) oxidation at a polycrystalline Pt electrode. The spectra are displayed as potential difference spectra with bands pointing upwards representing the reference potential (-0.1 V vs. Ag/AgCl/KCl (sat)) and downward bands belong to the indicated sample potential. Bands for reactant depletion and product formation can be clearly distinguished in the lower wavenumber region together with bands for CO2 formation indicating total oxidation (2341 cm-1) and adsorbed CO at about 2060 cm-1 indicating dissociative adsorption of ethylene glycol at Pt.
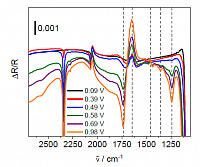
IR difference spectra of ethylene glycol oxidation at a polycrystalline Pt electrode in perchloric acid solution. From: “Spektro-elektrochemische Charakterisierung der Ethylenglycol-Oxidation an Platin- und Gold-basierten Katalysatoren“, Dissertation, Alice Schätz, Halle (Saale) 2021.
For the near future it is planned to extend this research towards non-noble metal based catalysts.
Materials and methods for electrochemical CO2 reduction
The direct impact of CO2 emission rates on atmospheric temperatures has prompted scientific attention toward the development of innovative and sustainable materials cycles. Based on the increasing accessibility of abundant and cost-effective renewable energies, electrochemical CO2 reduction emerges as a powerful tool to convert CO2 into valuable products without relying on additional fossil energy sources. However, the technical CO2 electrolysis still encounters several challenges, in particular in terms of CO2 transport limitation in aqueous electrolyte solution, selectivity control for specific hydrocarbons or competition towards hydrogen evolution.
Various gold catalysts have been investigated for electrochemical CO2 reduction, since the synthesis of defined gold nanoparticles has proven to be reliable. As most of these materials are selective for CO evolution, the design of gold catalysts with unique properties has become an active field of research. This project aims to modify the electronic properties of gold nanoparticles by depositing them onto oxidic supporting materials such as Magnéli phases in order to manipulate the CO2 reduction towards hydrocarbon evolution.
One focus of our research is on structure-selectivity relationships. The supported gold catalysts will be comprehensively characterised using various methods like X-ray diffraction (XRD), scanning electron microscopy (SEM), X-ray photoelectron spectroscopy (XPS), UV/VIS spectroscopy and Raman spectroscopy. Furthermore, the potential dependent product distribution from CO2 reduction over defined catalyst materials will be analysed using gas chromatography (GC) as well as scanning electrochemical microscopy (SECM), while the reduction itself is monitored with basic electrochemical methods such as chronoamperometry (CA) or linear sweep voltammetry (LSV). Ideally, dynamic processes like structural transformations of oxidic phases or surface adsorption of intermediates can as well be clarified using in situ techniques based on Raman spectroscopy or infrared spectroscopy.
Novel in situ-methods in electrocatalysis research
Electrochemical energy conversion plays a key role in a future renewable energy scenario, especially in the field of energy storage. The main components of electrochemical energy conversion devices are the electrodes, which should have both high activity and high stability, at a reasonable price for a commercial application. In situ-electrochemical techniques are required for a better understanding of the processes occurring at these electrodes.
Vibrational spectroscopies, such as infrared (IR) and Raman spectroscopy, are used in our group as in situ-techniques to study product or intermediate formation, phase transitions or structural degradation phenomena. Additionally, surface enhancement techniques (SEIRAS, SERS) are also implemented into the experimental procedures and well-known methods are modified or adapted so that they can be used to analyze the most important electrochemical conversion reactions.
Furthermore, position-resolved electrochemical techniques are well established in our group and can be modified for any conceivable application. Scanning electrochemical microscopy (SECM) has been widely used to characterize various electrochemical conversion reactions at numerous active structures and materials by applying a microelectrode as probe. In case of scanning electrochemical cell microscopy (SECCM), a micropipette is used as a type of confined reaction volume that can probe various spots at a surface. New methods are developed for both techniques depending on the material to be examined (electrode, electrolyte, active battery material) and both can also be combined with Raman microscopy to gain further complementary structural information.
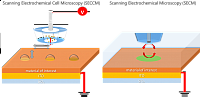
In situ methods