Kontakt
Prof. Dr. Martin Weissenborn
Telefon: +49 345 55 21660
martin.weissenborn@chemie.u...
Raum A.2.10
Weinbergweg 22
06120 Halle (Saale)
Research in the Weissenborn Lab
Übersicht
New research themes in the Weissenborn Group
A few new exciting research projects and themes that are currently being developed in the Weissenborn group are as follows:
- Performing mutagenesis on the Ribosome.
- Tailoring enzymes to perform polymer degradation
- Using machine learning to aid enzyme design.
Biocatalysis
In catalysis, there are metallo- and organocatalysts. Another field of catalysis is biocatalysis. In biocatalysis, natural proteins are employed to catalyze chemical reactions. In our body and every living organism, thousands of reactions occur simultaneously, and most of them are catalyzed by proteins. Catalytically active proteins are enzymes. The complexity and necessity of precision work in the living organism already illustrate the strength of enzyme-catalyzed reactions: unmatched selectivity, i.e., substrate specificity, and the regio-, chemo- and stereoselectivity of the conversion. Additionally, enzymes are produced from renewable sources, are biodegradable, and perform in water at a neutral pH.
We aim to use our chemical knowledge to use enzymes for chemically challenging or even impossible reactions.
Directed Evolution
Although nature holds an impressive diversity of available enzymes, often the enzymes need to be “adapted” towards the property of interest. In nature, the enzymes have developed in millions of years towards the activity, stability, or even catalysis required for the cell survival: the Darwinian Evolution.
Performing this evolution in the laboratory has been recognized with the Nobel Prize in Chemistry 2018 for Frances Arnold and is called Directed Evolution. Please have a look at her Nobel Prize lecture to get some insights here . In the figure below, the general workflow of directed evolution is shown. The gene which corresponds to the protein of interest is mutagenized by random or semi-rational means. The generated mutant library is transformed into cells that express the genes, thus producing the corresponding proteins. The variants are then screened for an altered function like increased activity, changed selectivity, or stability. The scientist decides in which direction the enzymes will be evolved. Hence the term: Directed Evolution.
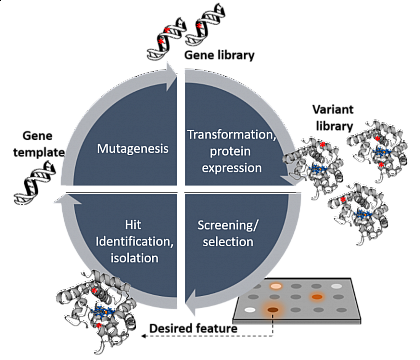
Lit: PhD thesis of Anja Knorrscheidt, MLU Halle-Wittenberg, 2021.
Mutagenesis
For the facile creation of site-saturation libraries at several positions simultaneously in one step and one day, we have developed a Golden Gate-based technique called Golden Mutagenesis (Scientific Reports 9, 2019 ). Golden Mutagenesis is exceptionally efficient, leading to up to 100 % correctly assembled plasmids. With Steffen Neumann’s group, we designed an online tool, allowing for an easy and rapid primer design. The sequencing results can be uploaded to determine the diversity of the created library. Feel free to use it. See here . All developed plasmids are available at AddGene .
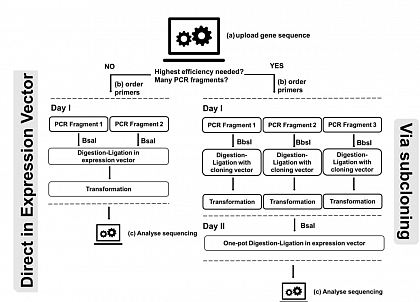
Lit. Scientific Reports 9, 2019
Screening
The bottleneck of all directed evolution endeavors is the screening of the protein variant library. Many techniques to rapidly assay large libraries have been developed. However, most of them are enzyme or substrate/product-dependent. We have developed a versatile high throughput GC-MS technology called MISER GC-MS, which allows the analyte measurement every ~30 s (ChemCatChem, 12, 2021 ). Since the detection is by MS, we have further expanded the system to screen for up to six products in parallel – some only distinguished by different fragmentation patterns. This approach allowed us to screen a small library of only 900 transformants to see variations in substrate specificity, activity, and regioselectivity (Cat. Sci. Technol., 11, 2021 ).
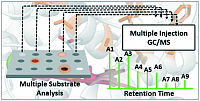
Lit. Cat. Sci. Technol., 11, 2021.
Exciting new enzymes and reactions
As the directed evolution requires several cycles and the expression of thousands of different mutants, most protein engineering campaigns rely on E. coli as a simple expression host. However, this focus on a prokaryotic host restricts the enzyme diversity. Several animal, plant, and fungus-derived enzymes are post-translationally modified and not or barely producible in E. coli.
The yeast Pichia pastoris can perform post-translational modifications and has proven to secrete proteins on an industrial-relevant scale. We developed a modular secretion and detection system, enabling the screening of large enzyme libraries in Pichia pastoris. The screening was performed with an exciting C–H activating enzyme class: the unspecific peroxygenases (UPOs). An enzyme class, which can perform hydroxylation reactions of non-activated sp3-hybridized carbons. However, only one variant could be heterologously produced in yeast when we started the project.
Modular yeast secretion technique
We developed a Golden Gate-based system, which enabled the rapid screening of different parts of an expression/secretion cassette and started with Saccharomyces cerevisiae as the more established yeast organism. This cassette consisted of a signal peptide responsible for the secretion, different UPO genes, and a C-terminal-tag responsible for protein purification and detection via split-GFP. With the Golden Gate principle, we were able to identify the ideal C-terminal tag and the best signal peptide/UPO gene combination and were able to secrete seven out of the tested eight UPOs (Commun. Biol. 4, 2021 )! All developed plasmids are available at AddGene .
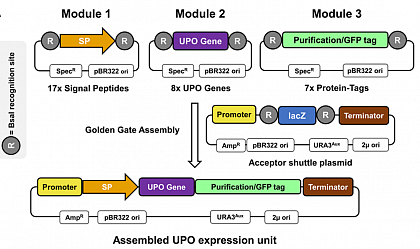
Lit. Commun. Biol. 4, 2021.
Directed Evolution of Unspecific Peroxygenases UPOs
With the new system to access and screen new UPOs, we utilized the MthUPO from a thermophilic fungus to perform directed evolution. The aim was to create chemodivergent variants, where one can hydroxylate at the benzylic position and the other oxyfunctionalizes the aromatic ring (ACS Catal. 11, 2021 ). Initial single site-saturation libraries were created using Golden Mutagenesis. All hits were recombined in an extensive library. The screening of more than 5000 transformants resulted in the identification of the selective variants. The aromatic oxyfunctionalization produces the important compound vitamin K3 (collaboration with Marc Garcia-Borras).
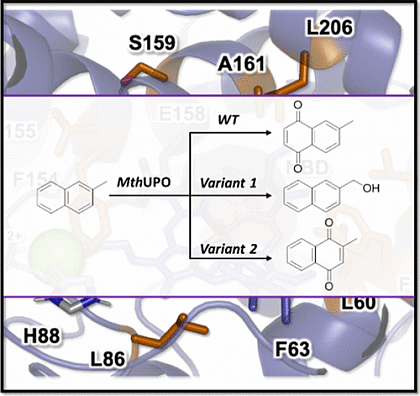
Lit. ACS Catal. 11, 2021.
Expansion of the system to Pichia pastoris
To adapt the system to P. pastoris, an episomal and integrative vector P. pastoris vector was designed. The episomal system has higher transformation efficiency, less interclonal variability, and easier sequencing, whereas the integrative system is more stable. In P. pastoris, by far, most work has been done using genomic integration concepts. Both systems were tested, resulting in the successful secretion of a range of UPOs using two different promoters.
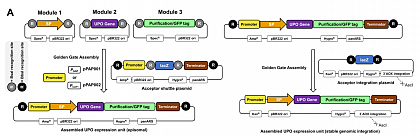
Lit. Commun. Biol. 4, 2021.
Preparative scale UPO conversion
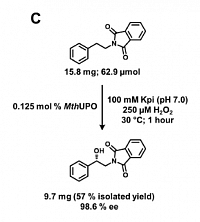
Lit. Commun. Biol. 4, 2021.
The new P. pastoris system resulted in improved production titers of several UPOs. With these titers, sufficient amounts of the enzyme could be isolated to perform preparative scale conversion of important phenethylamine-derivatives and isolate the enantioselectively hydroxylated product (Commun. Biol. 4, 2021 ).
Promoter screening in Pichia pastoris
The previously developed episomal expression system in P. pastoris has the advantage that large libraries can be screened. We noticed that the combination of the promoter and the signal peptide has a tremendous and not predictable influence. We expanded the tripartite system with a fourth module: promoters. 11 different promoters from P. pastoris and Hansenula polymorpha were screened in combination with the 17 different signal peptides. The screening resulted in several cases of substantially improved secretions for UPOs, lipases, and laccases. Also, the secretion of three further UPOs was described for the first time in yeast (ACS Synth. Biol. 10, 2021 ). All developed plasmids are available at AddGene .
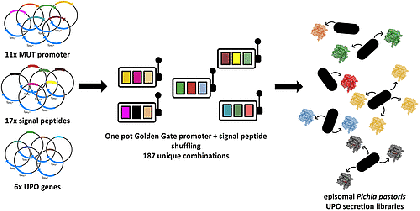
Lit. ACS Synth. Biol. 10, 2021